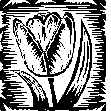 |
Plant Physiology (Biology 327)
- Dr. Stephen G. Saupe; College of St. Benedict/ St.
John's
University; Biology Department; Collegeville, MN 56321; (320) 363 - 2782;
(320) 363 - 3202, fax;
ssaupe@csbsju.edu |
Plant Positioning Responses (or Guidance Systems)
I. Need for Positioning
Recall our "plant way of life"
discussions. We concluded that non-motile organisms, such as plants, have a
limited ability to position themselves in their environment since they cannot
move to a more favorable environment. The major positioning responses used by a
plant, in approximate order of need, are: (a) environmental positioning - mostly
by chance/luck (i.e., seed germination mechanisms); (b) axis orientation -
gravitropism; and (c) fine tuning mechanisms- assorted responses to maximize
environmental resource acquisition and utilization (i.e., phototropism). Here we
focus on axis orientation and fine-tuning responses, which require the plant to
"move," at least to a small degree.
II. Movements. Plants exhibit three major types of movements:
- Hydration movements
These result from the uptake of water by non-living
cells/tissues and is responsible for actions such as the opening of a fern
sorus, the opening of some types of fruits (i.e., witch hazel,
wild cucumber), and the twisting of awns in porcupine grass (Stipa) and
other grasses;
- Turgor movements
Reversible; responsible for phenomena such as sleep movements (nyctinasty)
and movement of leaflets of the sensitive plant; and
- Growth movements
Irreversible; result from differential growth
(elongation). There are several kinds of growth movements including: (1)
tropisms - unidirectional responses that are related to the direction of
stimulus (positive - toward stimulus; negative away). Examples include
phototropism and gravitropism; (2) Nastic responses - in this case the
response is not related to the direction of the stimulus (i.e., thigmonasty,
epinasty, seismonasty); and (3) Nutations - rotary type movements of plant
structures, particularly the shoot tip (i.e., circumnutation).
III. Responding to the environment
Plants respond to signals from their environment. These
signals are received by the plant and then converted into a physiological
response. This sequence of action can be diagrammed as follows: environmental
signal → receptor
→
transducing mechanism (includes amplifying the signal) → physiological
response.
- Signals
Plants respond to many stimuli or signals in their
environment. These include light, wind, and gravity. Overall, light is one
of the most important environmental signals and it is involved in many
different responses. Photomorphogenesis is the fancy term for a plant growth
response to light.
- Receptor
An environmental signal must be intercepted or received
by the plant. The nature of the receptor depends upon the stimulus. The
receptor for any light-induced stimulus is a pigment, which by definition is
a molecule that absorbs light.
- Transducing mechanism or chain
This refers to the sequence of events by which the
activated receptor converts the signal to a physiological response. The
mechanism generally requires: (1) an amplification of the signal; and (2)
the plant must be in a physiological state to be able to respond to the
stimulus.
IV. Gravitropism
A. Stimulus - gravity, hence the name gravitropism. It used to be called
geotropism, but gravitropism is more accurate since the response isn�t toward
the earth ("geo")
- The response varies - thus, the gravitropic response may be parallel to
the direction of the stimulus (i.e., roots and shoots, called
orthogravitropic), perpendicular to the stimulus (diagravitropic, as in
rhizomes and stolons) or at an angle other than perpendicular or parallel
(called plagiogravitropic; i.e., lateral roots)
- How to study gravitropism on earth, especially considering there can�t
be a "no gravity" control? One answer - a clinostat. This device is
essentially a phonograph turntable to which a plant is attached. The
entire unit is held horizontally and the plant rotated to counteract the
effect of gravity.
- The threshold intensity - the minimum amount of stimulus required to
induce the response. Roots are more sensitive than shoots. Oat coleoptiles
in clinostat experiments respond to 0.0014 g, whereas roots require
just 0.00014 g.
- The threshold dose - represents the minimum dose of
stimulus required to induce a response - it equals the product of stimulus
intensity times the duration of the stimulus. The threshold dosage for oat
coleoptiles varies from 30-240 g s.
- Presentation (or reaction) time -
minimum time necessary to induce a response which can be as short as 12
seconds for cress roots. Usually there is a lag of about 10 minutes
between the time of the dose and the response.
B. Receptor
- Does one exist?
You bet. Plant on side
→
place upright → bends. One conclusion: the
sites of stimulus and perception are separated.
- Location in root?
Root cap. Decapitated roots don�t respond to gravity.
Interestingly, gravity seems to be necessary for the regeneration of the
root cap in Zea mays - when decapped in microgravity (on the Space
Shuttle) maize roots don�t regenerate a cap. This observation suggests
that a part of the root other than the cap also perceives gravity.
- Location in shoot?
Not known precisely, though it seems to be associated
with a starch sheath in stems, such as sunflower hypocotyls and the
dandelion scape (flowering stalk). Peeled
hypocotyls don�t respond to gravity suggesting the epidermal layers are
important in sunflower. The coleoptile tip is most responsive - but, even
if you remove the tip, coleoptiles still show some sensitivity to gravity.
- Nature of the receptor - statoliths
These are small bodies that have a high specific
gravity and presumably settle to the bottom of cells (statocytes) to
"tell" the plant which way is down. Statoliths are probably
amyloplasts (or starch grains). The evidence for this conclusion is: (a)
Presentation time - there is a correlation between the rate of amyloplast
sedimentation and presentation time; and (b) GA or kinetin treatment at
elevated temperature (35 C), which causes starch to disappear in the roots
of cress seedlings, abolishes the gravitational response. Recovery of
gravitropism is correlated with the reappearance of amyloplasts.
Specifically, which cells in the root cap are the
statocytes? The root cap has three layers of cells: (a) calyptrogen (which
are meristematic producing new root cap cells; (b) columella cells (named
for their shape; filled with amyloplasts); and (c) peripheral cells (secrete
mucilage). Thus, the columella cells are the likely statoliths.
- Nature of the receptor - Protoplast
buoyancy? Stretch Receptors?
One major problem with the statolith hypothesis is that
starchless mutants of Arabidopsis are still gravitropic. This
inconsistency (and others) have led to the suggestion (see the series of
three articles by Staves, et al., in the American Journal of
Botany, volume 84, pp. 1516 and 1533, 1997) that buoyancy of the
protoplast is the means by which plants sense gravity. Recall our
Chardokov experiment in which dye drop either sank, floated or hovered,
depending upon the density of the medium. Thus, if the protoplast is
suspended in a less density medium, like air, it will sink and press on
the bottom of the cell. Similarly, in a more dense medium, it will float
and press on the top of the cell. Using the green algae Chara,
Staves and colleagues observed that incubating the cells in media of
different density affected the gravitropic response as predicted by the
buoyancy model. They further hypothesized that when the protoplast
contacts the wall it stimulates proteins (called integrins) which lead to
a transduction response.
C. Physiological response
Proton efflux on upper side of a gravistimulated root (and
lower side of the shoot) causes the upper root cells to be more plastic than the
lower ones. Thus, these elongate more and shoots bend up and roots bend down.
This is rather similar to the way a vehicle with tracks, like a tank, turns - one tread
remains stationary while the other continues along (Mulkey et al. - data
from acid efflux experiments with maize roots).
D. Some observations
- The response is fast - the lag or presentation time is 7-60 min. Amyloplasts fall in about 12 sec;
- A signal is transmitted from the site of perception (root cap) to the
zone of elongation where bending occurs;
- A growth inhibitor in the root or growth promoter in the shoot may be
involved (see data);
- Cholodny/Went hypothesis - suggested that auxin accumulates on the
lower side of gravistimulated tissues. The increased auxin content of the
stem stimulates elongation of cells on the lower side but inhibits roots;
- Placing a root on its side results in change in electrical potentials at
the tip (see data);
- There is a calcium gradient in gravistimulated roots and shoots - (a) in
shoots there is more calcium on the upper side, but more on the lower in
roots; (b) in roots, calcium moves through the mucilage. If you remove the
mucilage by washing, no response (see data). Further, aquatic plants have
non-responsive roots; (c) the asymmetrical distribution of calcium can
cause bending (see data); (d) EDTA binds (removes) calcium - bending away
from EDTA application; and (e) IAA moves toward calcium (Ca2+ )
- Roots show electrotropism - curve toward the anode (AJB 77:446, 1990).
- Auxin is required for graviresponsiveness
- IAA is laterally transported downward in some tissues like
maize coleoptiles there are conflicting reports about the presence of an
IAA gradient across shoots and roots.
- SAURS - small auxin up-regulated RNA's; within
20 minutes these accumulate on the lower side of a horizontal stem
D. The transducing mechanism
Plant on side → amyloplast (or
protoplast) settles → associates with
ER/microfilaments/proteins or wall → may stimulate calcium
secretion and calmodulin → stimulates electrical
gradient → redistribution of Ca2+ secreted
by peripheral cells → calcium moves in mucilage
toward tip → auxin follows (calcium acts as a
sink) or calcium sensitizes tissue to the presence of auxin →
stimulates proton pump → increases cell wall
plasticity
→ unidirectional growth.
V. Phototropism
Growth response to unidirectional light or a gradient (more
on one side than the other) of light. Some of the first studies by Darwin (Power
of Movement in Plants, 1881). Plant response to light varies (shoots -
positively phototropic; roots - non-phototropic; leaves - plagiophototropic).
Some change during development (i.e., juvenile ivy - negatively phototropic,
adult - positively phototropic; Cymbalaria muralis - stems negatively
phototropic after fertilization to "bury" the pods).
A. Stimulus - light
- Which kind?
The action
spectrum suggests blue light (two peaks near 475 and 450 nm, and one in UV-A
at 370) � a "three finger response."
- Dose response
Which is more important: (a) fluence rate (μmol m-2
s-1); (b) duration (time) of the exposure (sec); or (c) the
total exposure (also called fluence, μmol m-2 ) which equals
the exposure multiplied by the duration?
For example, let�s assume that a 10 sec treatment
with 1.4 μmol m-2 s-1 blue light yields a curvature of
15 degrees. Thus, the total exposure is 14 μmol m-2 (= 10 s x 1.4
μmol m-2 s-1). If the plant is responding to the total
exposure, then as long as we provide a total exposure of 14 μmol m-2,
we should be able to vary the fluence rate and/or duration to yield the same
results. Thus, a 100 sec treatment with 0.14 μmol m-2 s-1
light
should yield the same response.
Another way of looking at this:
Fluence rate
and time are reciprocals (Law of Reciprocity) of one another if the system
is proportional to total exposure. Thus, if reciprocity holds: (a)
increasing the duration of the exposure and decreasing the fluence; OR (b)
decreasing the duration and increasing the fluence - should both yield the
same response.
Does it? Yes, but....only at low total exposures. If
phototropic curvature vs. log fluence (μmol m-2) is plotted the
response is complex. Essentially there are two (or more) peaks. The first
peak is termed the first positive curvature and it can be followed by a
second peak (second positive curvature) and so on. Reciprocity only holds
true for the first positive curvature (at low fluence).
So, what does all this mean? These data suggest that
light does two things: (a) it acts as a trigger; and (b) decreases
subsequent sensitivity
B. Receptor
- Location
Shoot tip,
young leaves. Evidence - remove the tip of coleoptiles and no response (see
data).
- Nature of the receptor
Light responses are perceived by pigments. The action
spectrum suggests a yellow (red) pigment is involved (see data). To
determine which pigment plot absorption spectra of potential candidates to
find match with action spectrum. Two candidates: riboflavin and/or
carotene.
Riboflavin is the probable receptor because: (a)
carotene doesn�t absorb much below 400, but riboflavin does; (b)
carotene-less mutants still phototropic; (c) plants treated with fluridone
and norflurazon, which inhibit carotene synthesis, are still phototropic;
(d) flavin inhibitors, like potassium iodide or phenylacetic acid, reduce
phototropic sensitivity. But, the absorption spectrum of riboflavin is not a
perfect match - a combination of the two seems even better.
The actual pigment involved
is called phototropin. There are two phototropins (1 & 2). The
gene is called phot (it used to be called npt = non-photoresponsive
hypocotyls). Phototropin is a flavoprotein. There is a
gradient from tip to base, there the tip is more sensitive than the base.
Phototropin protein capable
of autophosphorylating protein kinases (add phosphates to serine/threonine
kinase). The N-terminal binds flavin, C-terminal kinase.
C. Physiological response
Sunflower seedlings mounted in agar with dye show proton
efflux from cells on the shaded side of the stem.
D. Observations
- Cholodny-Went hypothesis - light causes a
lateral redistribution of auxin to the shaded side and elongate more than on
the lighted side (see data). Evidence: (a) agar block experiments (see
data); (b) radioisotope redistribution experiments in maize (see data); (c)
elongation should be slower lighted side, faster on shaded because of auxin
redistribution - it is based on experiments in which small glass beads were
glued onto coleoptiles and photographed (see data).
- Blaauw Inhibitor hypothesis - an inhibitor is
synthesized on the lighted side of the stem. Evidence: (a) experiments in
which sunflower cotyledons are shaded; (b) a xanthoxin gradient is observed
in photostimulated radish and sunflower (see data). But there are some
contradictory data.
E. Transducing Mechanism
Blue light stimulates redistribution of auxin across stem to
shaded side resulting in increased proton efflux and hence elongation.
VII. Thigmomorphogenesis
Plant growth response to a mechanical stimulation such as
rubbing, wind, raindrops, etc. The termed was first coined by M. Jaffee.
Seismomorphogenesis is specifically the response to shaking.
Compared to unstimulated plants, mechanically-stimulated
plants: (a) grow more slowly; (b) increase more in diameter. In essence, they
are shorter and fatter. This response makes "sense" to minimize the
risk of breaking which is especially true for plants in the mountains. As an
example, compare plants grown in indoors (houseplants, greenhouse) with those
grown outdoors.
This phenomenon is due to ethylene (the triple response)
for the following reasons: (a) ethylene concentrations increase in response to
mechanical stimulation; and (b) ethylene treatment mimics these effects, i.e.,
inhibits shoot elongation and induce stem swelling.
mRNA synthesis is stimulated shortly after mechanical
stimulation. Four or five genes are activated, one of which is the gene for
calmodulin.
VIII. Seismonasty - sensitive plant (Mimosa pudica)
Leaves fold upon touch, or being burned or otherwise
stimulated. This response is very rapid, the plant responds within a second or
two. In addition, the stimulus can be transmitted to other parts of the plant.
The transmission occurs by:
- Electrical potential
The membrane is depolarized and generates an action
potential which is similar to that in an animal nerve cell, only slower. It
travels from cell to cell at the rate of about 2 cm/s. It may travel via the
phloem.
- Chemical factor
It is clear that one is released to move from one leaflet
to another. The substance must pass through vascular system. Ricca�s
Factor - substance isolated that induced response in other leaves. Turgorins,
gallic acid derivatives, are hormones that may give rise to action
potentials - like the animal neurotransmitter acetylcholine.
The action potential/chemical factor would stimulate rapid unloading of
potassium into the apoplast and the leaves fold.
IX. Nyctinasty - sleep movements
Many plants, especially legumes with compound leaves, fold up
their leaves at night. This may serve to: (a) prevent moonlight from inducing
phytochrome responses; or (b) conserve water/heat.
A. Stimulus - blue light
B. Receptor.
Is not known, but may be the blue-light absorbing pigment (cryptochrome)
such as in phototropism and/or phytochrome.
C. Transducing mechanism
Leaf movements arise when cells (called motor cells) in the
pulvinus, which is a swollen area at the base of the leaf and each leaflet,
undergo turgor changes. Swelling of motor cells on one side of the pulvinus
(extensor cells) and shrinking on the other (flexor) causes the leaves to open
"up" (perpendicular to incoming light). When the leaves "go to
sleep" or close "down", parallel to the stem, the reverse process
occurs.
The turgor changes are caused by the influx/efflux of
potassium ions through membrane channels, in a manner reminiscent of guard cell
opening/closing. Chloride ions also enter the pulvinus cells.
An ATP-requiring proton pump is apparently involved. The
pH of the apoplast decreases during potassium uptake. This perhaps establishes
an electrical gradient which drives the uptake of potassium.
Last updated:
04/24/2009 � Copyright by SG
Saupe