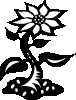 |
Concepts of Biology
(BIOL115) - Dr. S.G. Saupe (ssaupe@csbsju.edu); Biology Department, College of St. Benedict/St. John's
University, Collegeville, MN 56321 |
Primer on Biological
Energetics
I. Introduction to
Energy
What is energy? A simple definition - energy is the ability
to do work (which is the same as moving matter). Organisms need to do a lot of
work (i.e., metabolism, membrane transport, movement). Measured in
units of kJ (kilojoule) or kcal (kilocalorie - this is the traditional
unit and less preferred than kJ since it is a unit of heat. It is used
since all work can essentially be converted to heat)
- Forms of energy.
There are three biologically-important forms of energy:
(1) Chemical energy - energy involved in chemical bonds; (2) Electrical
energy - energy associated with electron flow; (3) Radiant energy - energy
that travels in waves and discrete particles (photons). Each may exist in a
potential or kinetic state.
- States of energy.
Potential energy is energy in a stored or inactive form;
energy of position (e.g., dynamite, standing on a desk, pulling back
a bow); Kinetic energy is energy in action (e.g., burning wood,
falling off a cliff, releasing the arrow).
- First Law of Thermodynamics.
Energy can be converted from one form to another, but
never created or destroyed. Or, to state it another way, the total amount of
energy in the universe is constant. Thus, plants adhere to the First Law
when, during photosynthesis, they convert solar (radiant) energy into
chemical energy. Similarly, during respiration mitochondria convert glucose
(potential chemical energy) into ATP (another form of potential chemical
energy) and heat (radiant energy). This process requires a flow of electrons
through a series of electron carriers (electrical energy).
- Second Law of Thermodynamics.
No energy conversion is 100% efficient. Or stated another
way, all systems tend to run down (because of inefficient energy
conversions). Or, all systems tend to a state of minimum energy, which is
the most stable state, which is a state of disorganization - called entropy
(symbolized by the letter S). It's no surprise that our rooms and offices
tend to get messy - because it takes energy to maintain things in an
organized state. In fact, all housework can be considered a battle against
the Second Law. Remember the Morowitz article, "Women's Lib and
the energy crisis?"
- Do organisms violate the laws of thermodynamics, especially the Second
Law, considering that organisms are highly organized?
No way. Life follows the Rules! To maintain an
ordered state requires a constant input of energy. Just like keeping a room
tidy requires a constant energy input, life requires a constant energy
input. Life is an open system - meaning it exchanges energy with its
environment (constantly replenishing energy needs). Life could not persist
isolated in a closed system (one that doesn't exchange materials with the
environment). Death can be considered loosing the battle to entropy
and metabolism can be considered the process by which life battles the
Second Law - the collective chemical processes by which energy is acquired
and utilized. Remember the Morowitz article, "Six Million Dollar
Man?"
II. Reducing Potential - ability of a substance to
participate in a redox reaction. Living organisms must carry out many redox
reactions.
A. Some definitions:
- Reduction - gain of electrons
- Oxidation - loss
of electrons;
- A helpful mnemonic: "oil rig" - oxidation is
loss, reduction is gain
- Redox reaction - reaction in which one
component is oxidized and the other is reduced. Obviously, electrons must come
from somewhere and go somewhere
B. The reduction sequence of carbon
carbon dioxide (most oxidized form of carbon) →
carboxyl (organic acid) → carbonyl (aldehydes,
ketones) → hydroxyl (alcohols) →
methyl → methane (most reduced form of
carbon).
Note: each step requires the addition (or removal) of two electrons and two
protons for reduction (oxidation). Two steps also require the addition of
water.
C. How can you tell if something has been oxidized or reduced?
- look for a change in valence (i.e., Fe2+
→ Fe3+ is an oxidation because an electron was lost,
increasing the total positive charge on the molecule);
- In many
biological redox reactions, oxidation is accompanied by a loss of protons
(hydrogen ions) and reduction is accompanied by a gain of protons. Thus, you
can count the number of hydrogen atoms on each side of the equation (the
more H, the more reduced); or
- count the number of oxygen atoms (the more O,
the more oxidized).
C. Biological redox reactions require electron donors and/or
acceptors.
These are usually: (1) NAD+ (2) NADP+
and (3) FAD. These are coenzymes (organic compounds, other than the
substrate, required by an enzyme for activity). The reaction sequence for
these coenzymes is given below:
NAD+ + 2H+ + 2e- � → NADH + H+
NADP+ + 2H+ + 2e- � → NADPH + H+
FAD + 2H+ + 2e- �→
FADH2
III. Chemical Potential - energy available from bond cleavage.
The primary source is ATP (adenosine triphosphate), which is
the energy currency of life. If a cell or organism wants to get some
"work" done, it "pays" for the work with ATP. It is
estimated that we use and cycle approximately a body weight worth of ATP
everyday.
- Hydrolysis of ATP to ADP + Pi releases energy used by cells.
eqn: ATP + H2O →
ADP + Pi + energy
- ATP hydrolysis is exergonic (ΔG = -7.3 kcal/mol).
- ATP hydrolysis is coupled to endergonic reactions.
Remember the hill model? ATP is what pushes the rock up
the hill. Since endergonic reactions are not spontaneous, the cell complete these vital
processes by coupling them to an exergonic reaction so that the sum of
the ΔG's for the two reactions is negative. Example:
A + B → C (ΔG = +4.0 kcal/mol)
ATP + H20 → ADP + P (ΔG = -7.3 kcal/mol)
A + B + ATP + H20 → C + ADP + P (ΔG = -3.3 kcal/mol).
Note that since the overall ΔG is negative, this reaction sequence is now
spontaneous and will occur. The actual "coupling" process is usually the result of ATP binding to one of
the starting materials. This essentially "energizes the reaction" making it
thermodynamically favorable. For more, check out the notes on energetics.
- ATP synthesis is termed a phosphorylation reaction (because a phosphate
group is added to ADP)
- ATP formation - substrate level phosphorylation
Occurs during glycolysis or the Citric Acid cycle. In a
substrate level phosphorylation the phosphate used to phosphorylate ATP
comes from an organic compound and the process is NOT associated with redox
reactions
- ATP formation - oxidative phosphorylation
Is the result of electron transport in the mitochondria
and chloroplasts. In contrast to substrate level phosphorylation, oxidative
phosphorylation uses inorganic phosphate and the process IS associated with
redox reactions via an electron transport chain (ETC).
IV. Equation Review.
Given that background, let�s review the equations for
photosynthesis and respiration that you�ve seen many times:
photosynthesis: |
CO2 + H2O + light
energy
→ (CH20)n + O2 |
respiration: |
(CH2O)n + O2
→ CO2 + H2O +
chemical energy |
Now, let's look at some exciting details!
- Photosynthesis and respiration are redox reactions.
During
photosynthesis carbon dioxide is reduced to a carbohydrate (which is
abbreviated as (CH20)n ) and the water is oxidized
to yield oxygen. Thus, the purpose of water in photosynthesis is to supply
the electrons for the reduction of carbon dioxide to a carbohydrate. The
situation is reversed for respiration.
- Photosynthesis is an anabolic and endergonic reaction. Light provides
the energy that is
required for the reduction of carbon dioxide.
- Respiration is a catabolic and exergonic reaction. There is a net energy
loss during the process. Some of the energy is used to make ATP.
- Hill Model Revisited - a diagram of the hill will be provided in class.
Some take-home-lessons from the hilltop:
- Anabolism is analogous to pushing the rock uphill, catabolism is
analogous to the rock rolling downhill;
- Photosynthesis (an anabolic process) is analogous to pushing the rock
uphill, respiration (a catabolic process) is analogous to the rock
rolling downhill;
- The energy required to push the rock (glucose) uphill comes from light
(radiant energy);
- The release of energy from glucose rolling downhill is coupled to ATP
production (ca. 40% of the energy is trapped in ATP but more than
half of the energy is lost as heat).
Last updated: August 20, 2004 Visitors to this site: 
� Copyright by SG Saupe / URL:http://www.employees.csbsju.edu/ssaupe/index.html