CHAPTER 9 - SIGNAL TRANSDUCTION
B: NEURAL SIGNALING
BIOCHEMISTRY - DR. JAKUBOWSKI
06/10/14
Learning Goals/Objectives for Chapter 9B: After class and this
reading, students will be able to
- describe how a transmembrane ion gradient and nongated/gated
membrane ion channels specific for given ions can give rise to a
transmembrane electric potential across membranes
- given ion concentrations and the electrical potential across
a membrane, predict likely changes in the membrane potential and
ion concentrations on the opening of specific channnels;
- use the Goldman equation to predict transmembrane electrical
potentials;
- state difference between the communication across the
neuromuscular junction and a synapse between two neurons;
- state the difference between nongated and gated ion
channels;
- describe different ways to open/close gated ion channels
- describe the immediate changes in the muscle cells when
acetylcholine is released into the neuromuscular junction
- describe the roles of stimulatory neurotransmitter
receptors, voltage-gated Na+and K+ channels and the Na/K-ATPase
in the activation of a neuron;
- explain the mechanism for selectivity of K+ over the smaller
Na+ ion in the K+ channel;
- briefly explain how membrane protein channels can be gated
open by changes in transmembrane potential;
|
B5. Proteins of the Neural Synapse
We must now account for the rise and fall of the membrane potential to a
variety of neurotransmitters, including the cholineric transmitters (ex.
acetylcholine), catecholamines (dopamine, epinephrine, norepineprine), amino
acid derivatives (ex. Glu, Asp, N-methyl-D-Asp, Gly, gamma-amino-butyric
acid -GABA), and peptides (endorphins, encephalins). We will consider five
membrane proteins:
Figure:
five membrane proteins
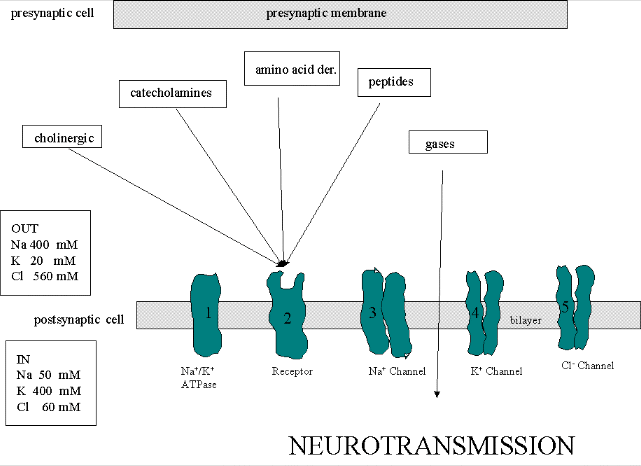
- Na+-K+-ATPase: We discussed this in the previous section on active
transport. It transports both sodium and potassium ions against a
concentration gradient using ATP as an energy source. The protein is a
sodium dependent ATPase. Without this protein, the membrane potential
could not be maintained since the sodium and potassium gradient would
collapse. It also contributes to the potential since its is an
electrogenic antiporter. (In addition, we have seen that ungated
potassium and sodium channels are also present.)
- Neurotransmitter receptor: The receptors we will consider here are
typically ligand-gated ion channels. Once ligand binds, a conformational
change occurs in the protein, allowing a flow of ions down a
concentration gradient. Depending on the nature of the ion, the channel
either initiates depolarization (when Na+ enters from the outside and
raises ΔΨ) or inhibits depolarization (when Cl-
enters from the outside and lowers ΔΨ). When chloride channels
open, they hyperpolarize the transmembrane potential. Stimulatory
neurotransmitters (like glutamate) lead to depolarization of the
membrane, while inhibitory neurotransmitters (like gamma-aminobutyric
acid) lead to hyperpolarization of the membrane (make the potential more
negative).
- Na+ channel (voltage-gated): When the ligand-gated channel
depolarizes the membrane to some threshold value, sodium channels
undergo a conformational change and open allowing Na+ ions to flood into
the cell, raising the potential to a positive approx. 33 mV (a value
lower than the the equilibrium sodium potential). This membrane protein
is a voltage-gated channel, not a ligand gated one. Somehow, it senses a
change in the transmembrane potential initiated by opening of the
ligand-gated channel.
- K+
channel (voltage gated): When the membrane potential reaches around
+25 mV or so, the K+ channel, a voltage-gated membrane protein, alters
its conformation, allowing K+ efflux from the cells, lowering the
potential until it reaches the potassium equilibrium potential. It
slowly relaxes back to the cell resting potential of about -60 mV.
Recently, the crystal structure of the K+ channel from Streptomyces
lividans was determined (Science, 280, 69-77, 1998): "All K+ channels
show a selectivity sequence of K+, Rb+ > Cs+, whereas permeability for
the smallest alkali metal ions Na+ and Li+ is immeasurably low.
Potassium is at least 10,000 times more permeant than Na+, a feature
that is essential to the function of K+ channels.....Two aspects of ion
conduction by K+ channels have tantalized biophysicists for the past
quarter century. First, what is the chemical basis of the impressive
fidelity with which the channel distinguishes between K+ and Na+ ions,
which are featureless spheres of Pauling radius 1.33 � and 0.95 �,
respectively? Second, how can K+ channels be so highly selective and at
the same time, apparently paradoxically, exhibit a throughput rate
approaching the diffusion limit? The 104 margin by which K+ is selected
over Na+ implies strong energetic interactions between K+ ions and the
pore. And yet strong energetic interactions seem incongruent with
throughput rates up to 108 ions per second."
- Cl- channel: If these channels (typically ligand-gated) are open,
they will hyperpolarize the cell and make it more difficult to fire.
Navigation
Return to Chapter 9B:
Neural Signaling Sections
Return to
Biochemistry Online Table of Contents
Archived version of full
Chapter 9B:
Neural Signaling

Biochemistry Online by Henry Jakubowski is licensed under a Creative Commons Attribution-NonCommercial 4.0 International License.